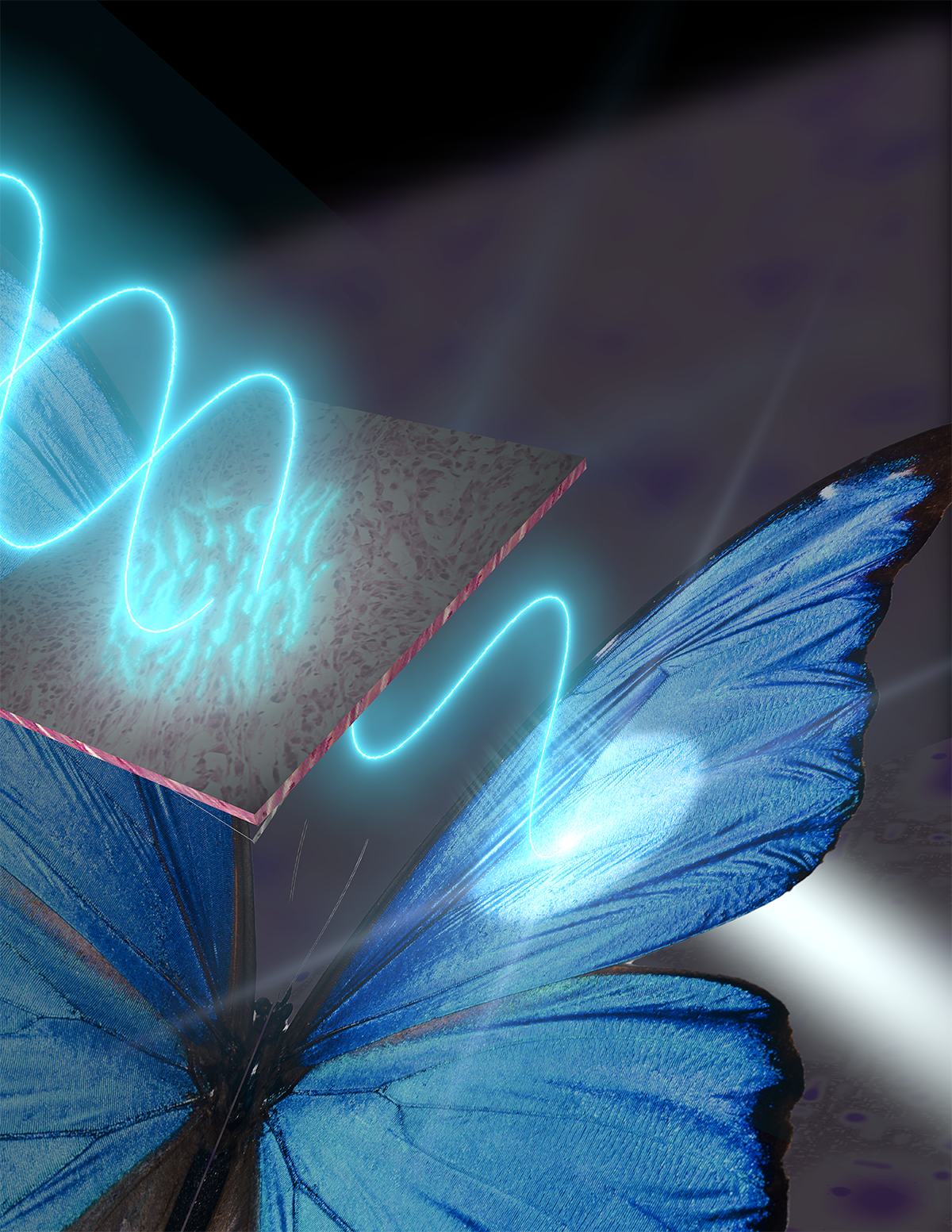
Nature-Inspired Photonic Surfaces for Imaging and Diagnostics
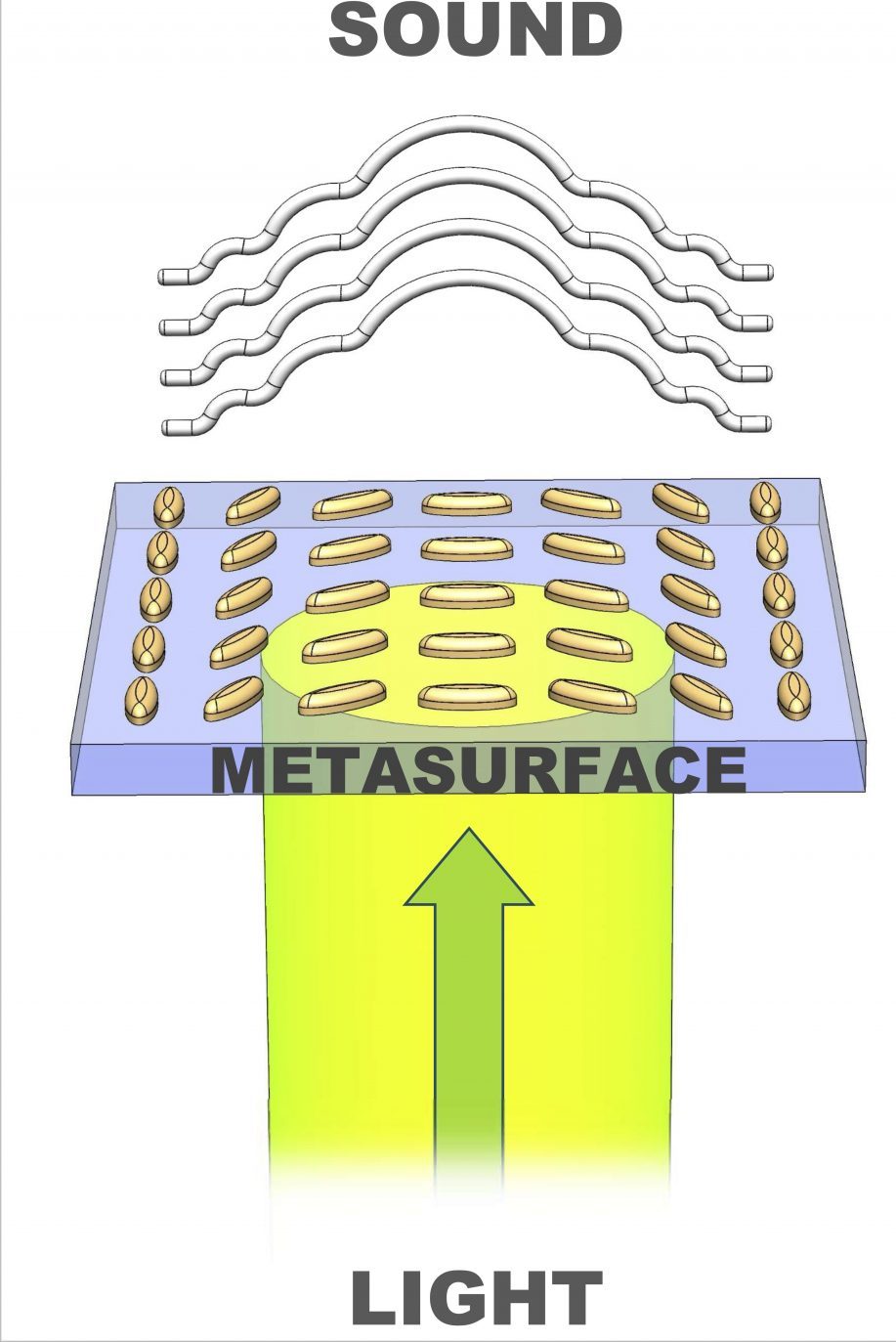
Acoustoplasmonics
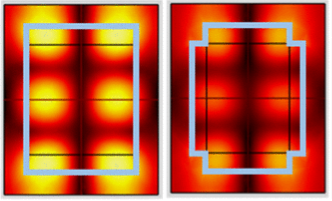
Chiral and Anisotropic Colorimetric Metasurfaces
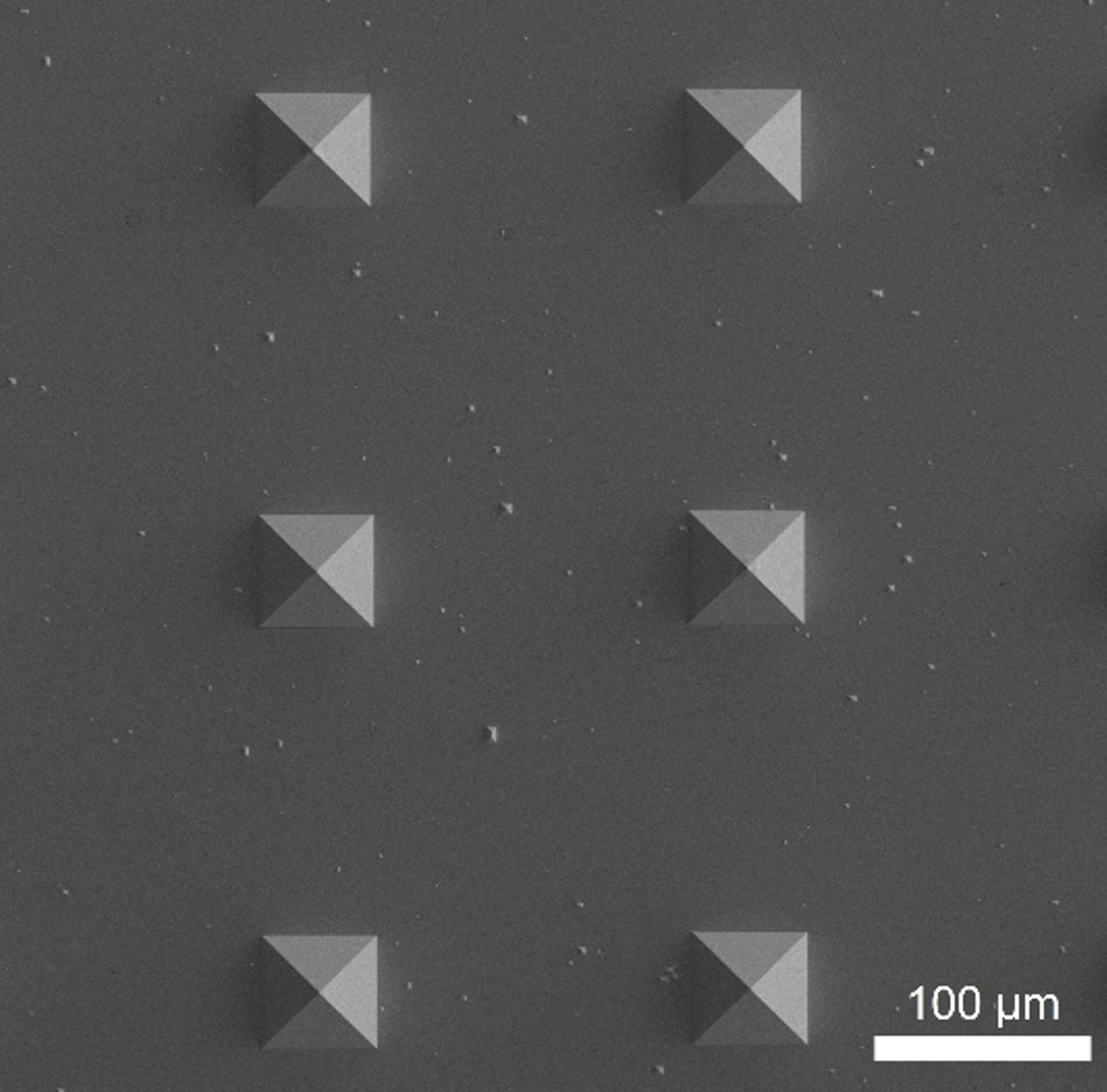
Laser Processing of Functional Photonic Materials
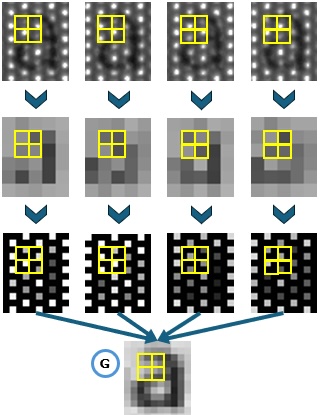
Structured Illumination
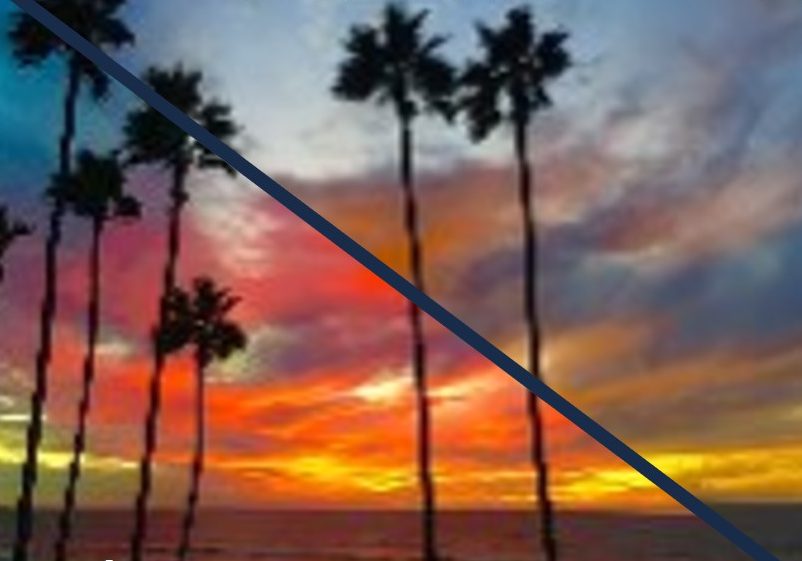
Spectral Filters and Recoloring Algorithms for Color Vision Deficiency
Addressing Biomedical Challenges with Novel Nanophotonic Probes
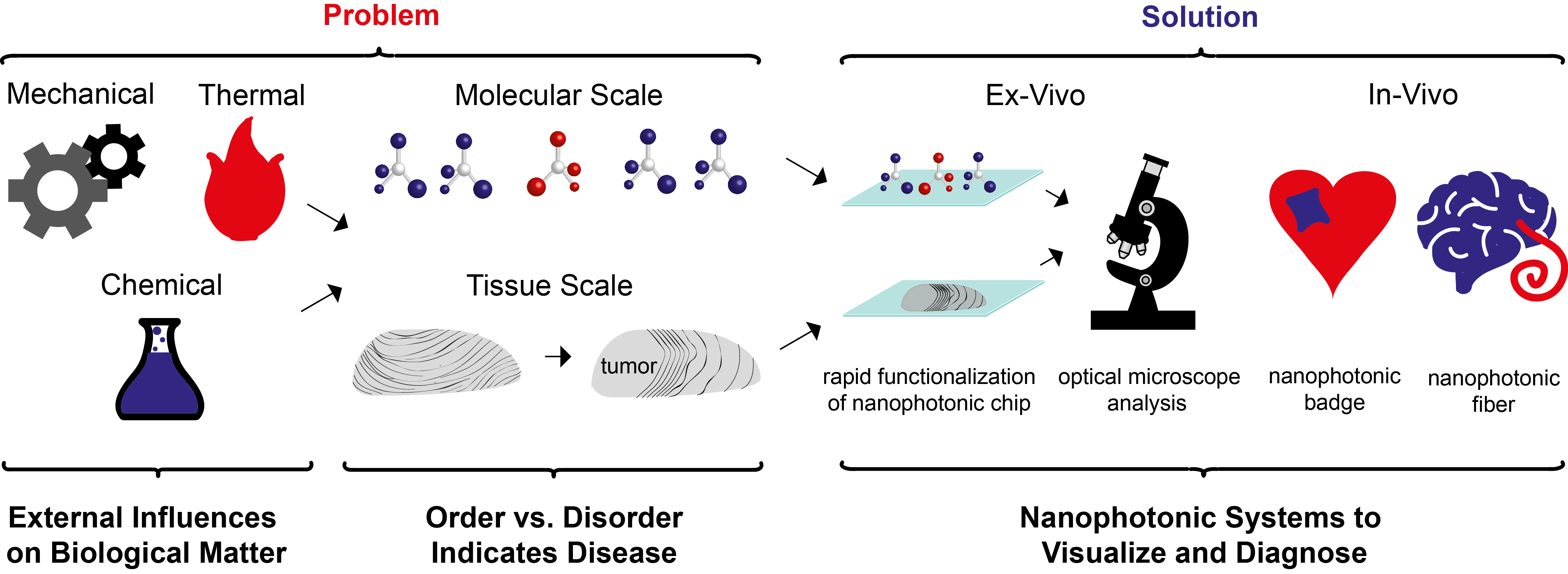
From the molecular to the macro scale, the interplay of order and disorder in biological matter is intimately linked to the onset and progression of leading global health challenges such as heart disease, Alzheimer’s disease and cancer. Anisotropic matter, ubiquitous in biological systems such as amino acids and ordered structural proteins, interacts selectively with light, thus presenting a rich palette of opportunities to non-invasively probe and visualize disease. However, naturally occurring anisotropic light–matter interactions, where light is an electromagnetic plane wave, are inherently weak, lying below thresholds relevant for biomedical applications. In the Poulikakos Lab, we address these challenges by leveraging the immense potential of nanophotonic materials to illuminate structural changes in biological matter for clinically-relevant disease diagnosis, assessment and quantitative optical visualization.
Nature-Inspired Photonic Surfaces for Imaging and Diagnostics
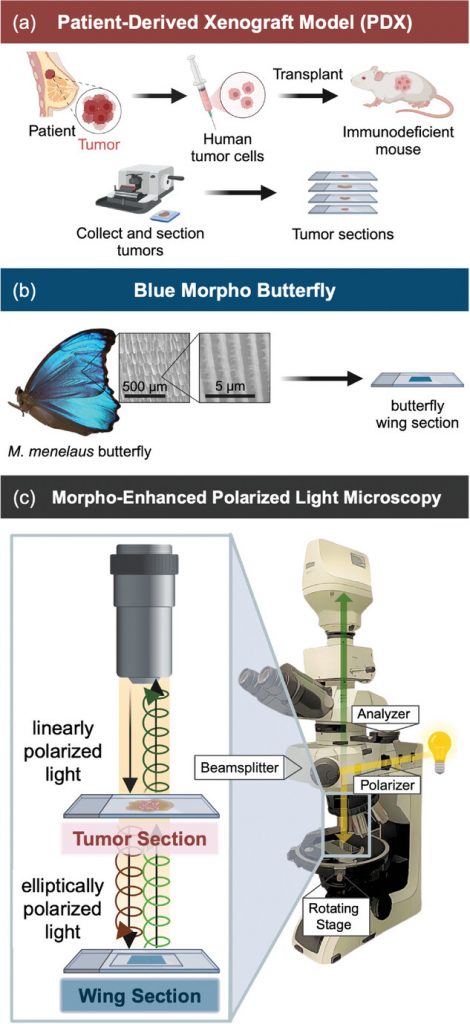
Throughout Earth’s history, organisms have characteristically evolved to persevere in their respective environments. Traits that enhance survivability and reproductive success are naturally selected, shaping the biodiversity we observe today. One astounding adaptation is the production of structural color, a phenomenon in which micro- and nanoscale features manipulate light to produce brilliant, angle-dependent colors. This property is intrinsic in certain butterflies, beetles, birds, and other organisms for purposes like attracting a mate or warding off predators. Many engineers interested in producing sensing technology have been working diligently to optimize the production of similar structures through synthetic fabrication. This is an often costly, complicated, and limited means to acquiring such advanced materials. We investigate a different approach – leveraging the sophisticated photonic structures found in nature for optical sensing. This is demonstrated in our novel imaging platform, Morpho-Enhanced Polarized Light Microscopy (MorE-PoL) which integrates the natural micro- and nanostructures of the Blue Morpho butterfly into a polarized light imaging platform. Not only do these features of the Morpho produce its brilliant color, but they are anisotropic; meaning their features possess different properties along different spatial directions. This results in an optical sensitivity to the incident angle or polarization of light. When interfaced with fibrous biological tissue – which changes the polarization state of light based on its microstructural properties – the butterfly wing translates tissue organization into distinct reflected colors. This enables stain-free, low-cost, and quantitative assessment of tissue microstructure, permitting the evaluation of many diseases.
Functional Metasurfaces for Rationally-Designed Light–Matter Interactions
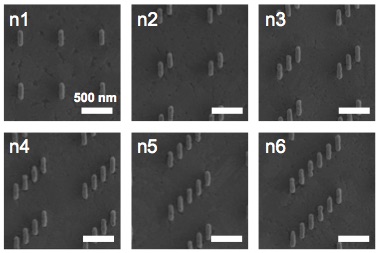
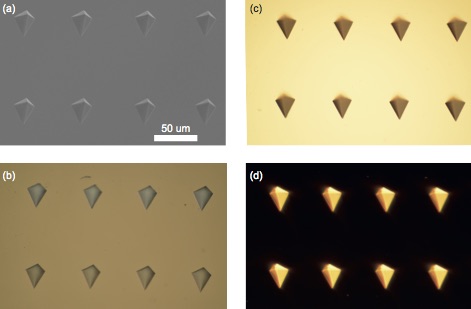
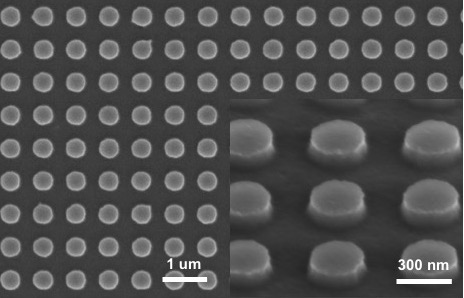
Metasurfaces are artificially engineered, two-dimensional systems which can alter electromagnetic fields in a manner unforeseen by nature. Such phenomena include negative refraction, electromagnetic cloaking and optical holography. In addition, these surfaces can be designed to replicate or enhance naturally occurring electromagnetic phenomena in a two-dimensional on-chip environment, including flat optical components such as metalenses and optically-thin waveplates. The key to metasurface design lies in the periodic arrangement of optical elements, known as meta-atoms, which are smaller than the wavelength of light. Meta-atom resonant properties can be controlled by their geometry and material composition. For example, meta-atoms exhibiting material or structural anisotropy enable a myriad of tailored interactions with polarized light. Their small scale enables the confinement of electromagnetic near fields to sub-wavelength dimensions and their two-dimensional periodic arrangement gives rise to strong collective resonances. In the Poulikakos Lab, we develop complex, on-chip optics with functional metasurfaces, thus opening new avenues to address technological challenges and create miniaturized, all-optical device architectures, including diagnostic applications with facile clinical implementation.
Chiral and Anisotropic Colorimetric Metasurfaces
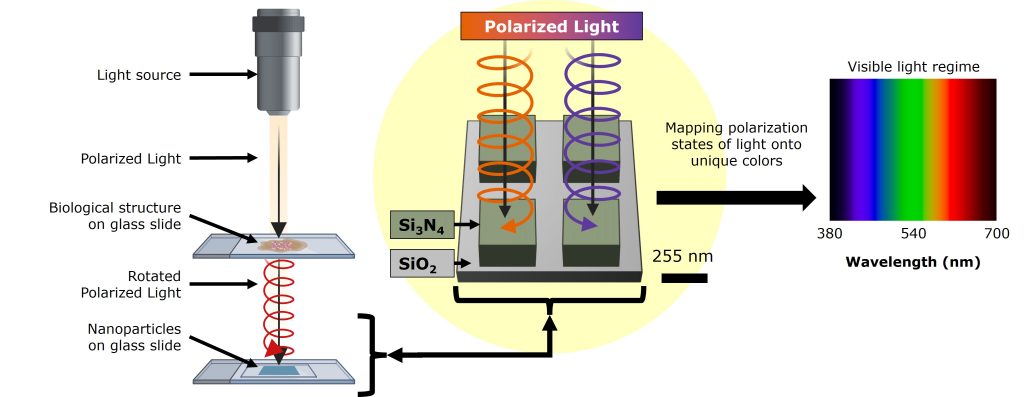
This project studies light-matter interactions for the purpose of enhancing light-matter interactions, because of biology. Biology made a beautiful observation: form dictates function. On the macro-scale, the fiber alignment in skeletal muscle is different from that in liver tissue; just as on the sub-cellular scale, the folded shape of a protein determines whether it carries oxygen or reads DNA. These structural differences – from tissue to protein – interact uniquely with polarized light, which is just a fancy way of describing the direction in which light wiggles: up-and-down, left-and-right (linear), in a circle (circular), or somewhere in between (elliptical). When light with a specific wiggle hits biological matter, the wiggle changes – but these changes are hard to detect with off-the-shelf microscopes and often require complex, meter-scale optical setups. We’re looking for a simpler route. Specifically, we design nanoparticles(!) whose shapes – like proteins – can be tuned to interact with specific light wiggles, enhancing the signal enough to be seen with a regular optical microscope. We’ve found that these particles can boost color contrast, purify the signal, and even tell apart left- and right-handed circular light – which helps us detect the handedness of chiral biological structures, a key feature of life. Our goal is to create a nanoparticle system sensitive to a wide range of polarizations, and to use it to distinguish between different biological forms. If successful, we can shrink meter-scale optical systems down to the nanoscale, enabling detection of biomarkers and biosignatures in resource-limited settings – on Earth or out in Space.
Laser-Processing of Functional Photonic Materials
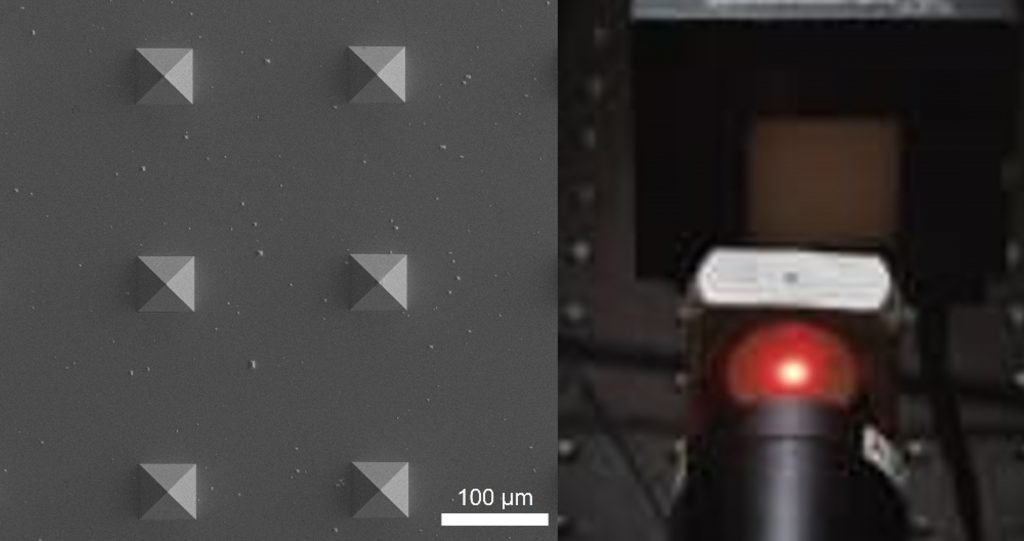
Laser-based techniques, categorized as additive manufacturing and ablation, are of interest to increase precision, speed, and quality in functional materials manufacturing. In photonics, systems miniaturization has been developed to exploit exceptional optical features in the nanoscale. Nonetheless, physical restrictions, such as the diffraction limit, hinder the feature size resolution. Therefore, we study the implementation of plasmonic systems for sub-diffraction limit laser focusing. Fabrication optimization based on template stripping has demonstrated ultrasmooth structures for enhanced localized plasmonic effect. Moreover, thermal robustness is strengthened by integrating refractory metals, including molybdenum, posing potential in high-power laser implementations.
Acoustoplasmonics
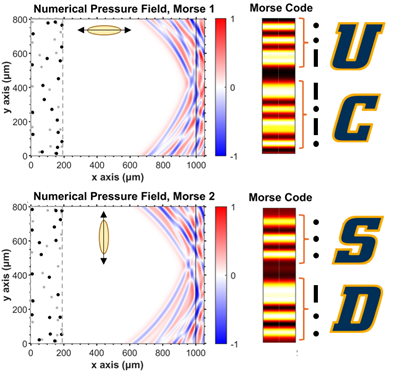
One branch of our lab’s research focuses on acoustic wave generation via acoustoplasmonics, in which plasmonic particles convert resonant optical energy into mechanical energy in the form of vibrations. We study photothermal heating of subwavelength plasmonic particles under pulsed laser illumination, which exhibit amplified light absorption under optical resonances – namely the localized surface plasmon resonance. This induces a photoelastic strain which launches a pressure wave. By exciting these particles at or near their acoustic resonance, we have the potential to generate ultra-high frequency waves. These mechanisms are studied both analytically and numerically, and soon to be studied experimentally. We have previously examined tunable photoexcitation using light polarization, and well as inverse design to intelligently arrange particles for complex wavefront generation. We envision applications in microfluidics for particle self-assembly, high frequency signal processing, and miniaturized acoustic imaging.
Structured Illumination
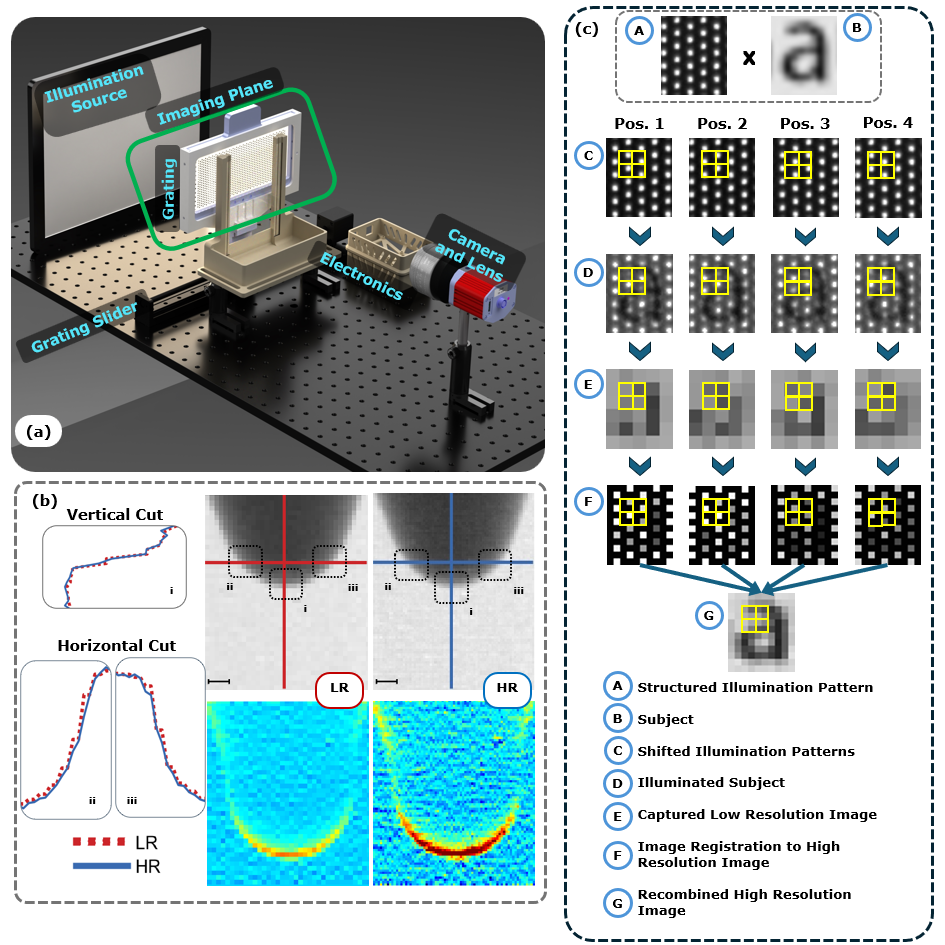
In flow imaging, intensity gradients are often a good measure of scalar gradients, which play an important role in controlling transport processes. However, camera pixel pitch limits the ratio between the largest to smallest scales that can be resolved in imaging. Furthermore, an increase in frame rates due to limitations in throughput reduces the maximum number of pixels that can be used for imaging. All these pose great challenges in the capacity to resolve small scales, affecting the estimation of gradients. Thus, it is of great interest to develop imaging techniques and post-processing methods that enhance the small-scale gradients in flow imaging. In collaboration with the Saha Research Group, we present a proof-of-concept demonstration of a new method and system inspired by Structured Illumination Microscopy (SIM) that can be used for flow imaging to enhance pixel resolution and, thereby, estimation of scalar gradients. This method utilizes sub-pixel-scale patterned light matching the system pixel scale and multi-frame imaging that creates quasi-static images over four frames with scalability for high-speed imaging. These multi-frame images are then processed using an in-house recombination algorithm that produces a new image with twice the pixel resolution compared to the original images. Part (a) of the figure shows the developed imaging platform, (b) shows how the illumination works with sub-pixel shifts and the recombination concept, and (c) shows an example of flow recombination with line cuts showing improved resolution in slopes and a first order gradient of the base images with an enhancement in pixel resolution.
Spectral Filters and Recoloring Algorithms for Color Vision Deficiency
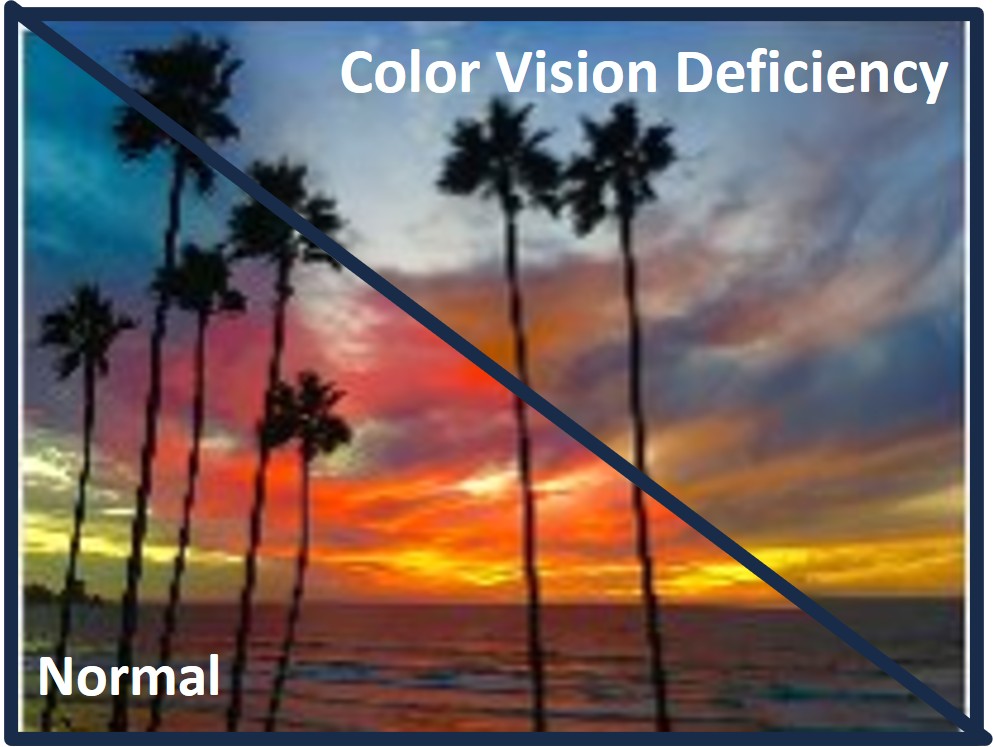
Color vision deficiency (CVD) results from a shift in the spectral sensitivities of cone cells in our eyes. Our team is building an algorithm to encode the type and severity of CVD to build spectral filters that increase color identification and distiniguishability. The team is also working on how to use both spectral filters and recoloring strategies, to optimize colors on digital displays for persons with CVD. This deep dive in color coding and perception helps us to understand color cameras and to simulate what colors a person with CVD sees to a person with normal vision. The current progress in the project is in how to incorporate metamerism and changes in illumination into the analysis workflow, and then how to quantify which spectral filter is optimal in maintaining color naturalness and consistency.
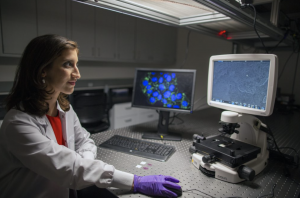
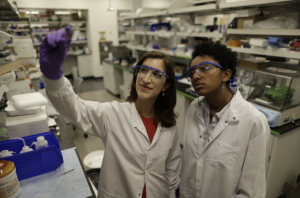